HI-Emission in nearby galaxies
6 november 2021
- -
- -
Spiral galaxies and their gaseous content
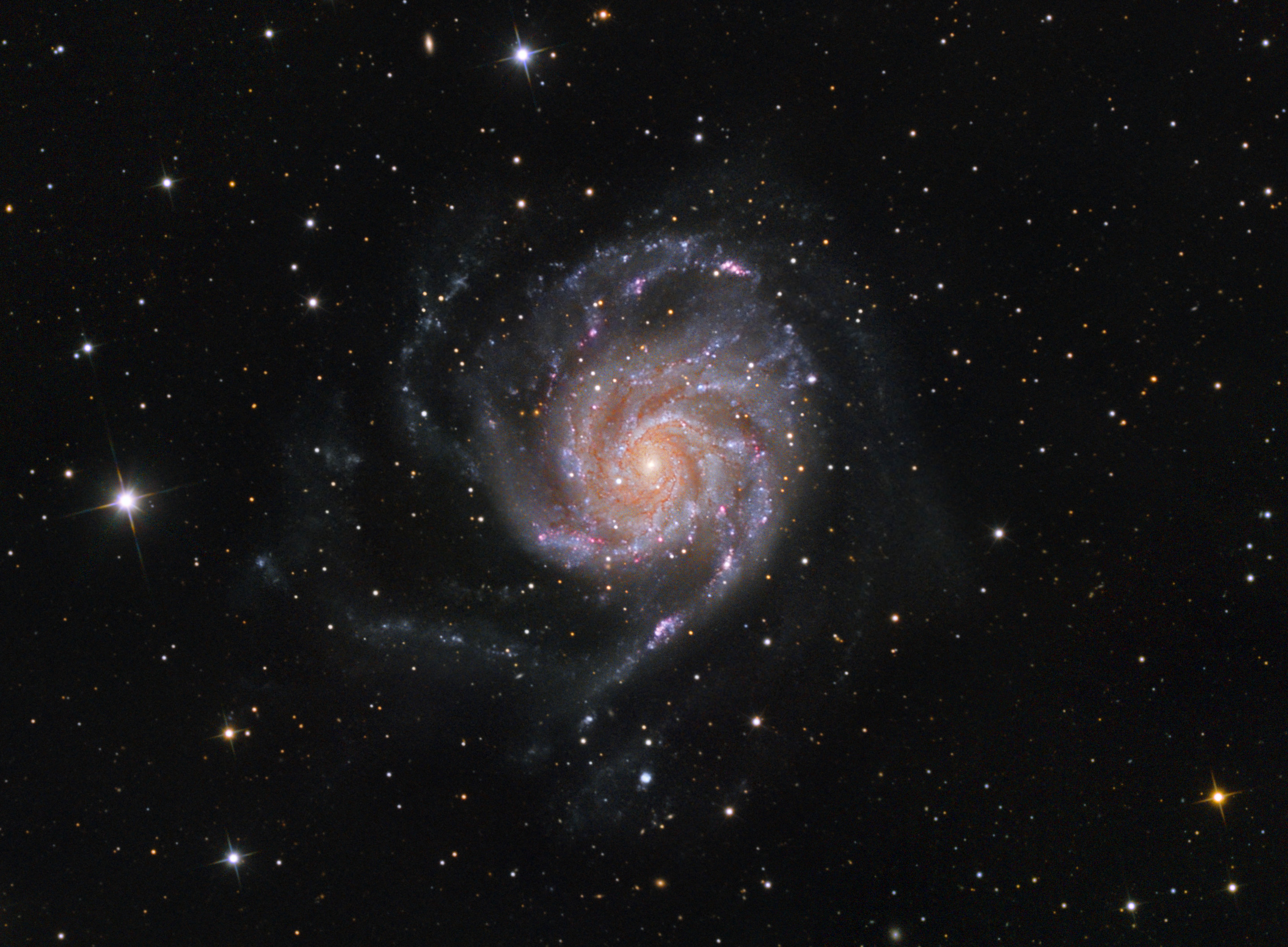
M 101
The main feature of a spiral- or S0 galaxy is its striking extended stellar disk, including dark lanes of cold molecular gas and dust, colorful emission nebula in active star formation regions and young blue star clusters. This diversity in colors, details and their different morphological features make them a popular and beloved class of objects for Deep Sky Astrophotography. Stars in the disk of a very large spiral galaxy, like our Milky way or M101 are the most important source of light in UV, visible and near-IR wavelengths. The reddish color of a galaxies bulge originates from an older stellar population, composed mostly of K-giant stars traveling in an unordered motion through this dynamically hot region free of of dust clouds and young star clusters. Most of the gas of a spiral galaxy is found in the disk; the cool atomic hydrogen “HI” and molecular hydrogen “H2” form the raw material out of which the galaxy makes new stars. Moving outward through the inner disk of spiral galaxies, we find prominent dust lanes of cold molecular gas which are home to active star formation. Cool gas can be seen directly by its emission in spectral lines at radio wavelengths, which propagate unhindered through the dusty disk. Young, bright blue star clusters can ionize the surrounding gas and let it glow in a characteristic red glow, the Hα-emission which defines these so called “HII”-regions.
But as we look further outwards in the disk, the density of stars and their brightness declines. However, as we see in the image below, where HI emission is overlayed over our image of spiral galaxy M101, the center is largely gas poor with a strong concentration of gas in the spiral galaxy’s disk, but the gas is spread out a lot further than the stars are. For this galaxy it extends to about twice the optical size.
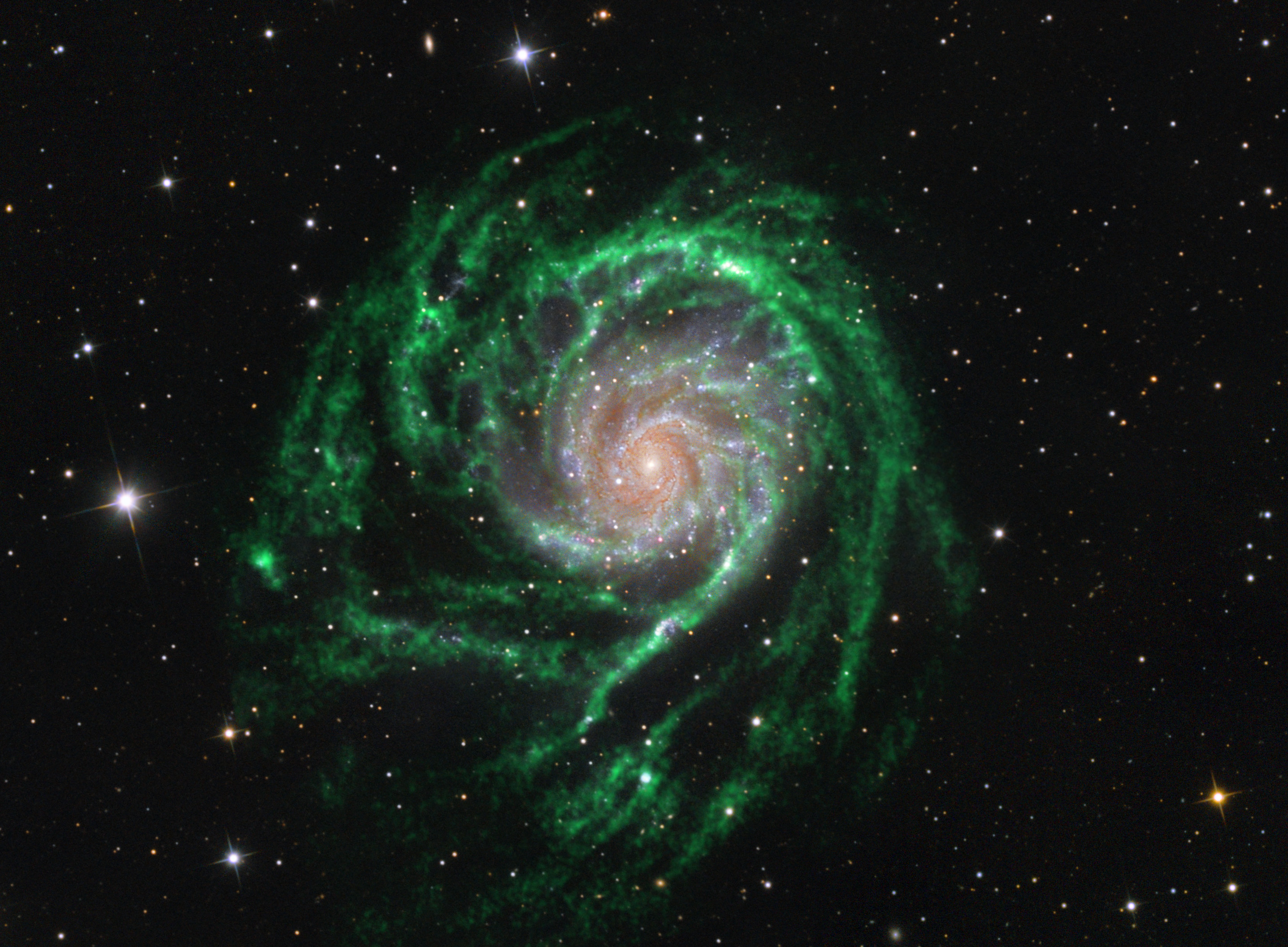
M 101 HI Overlay
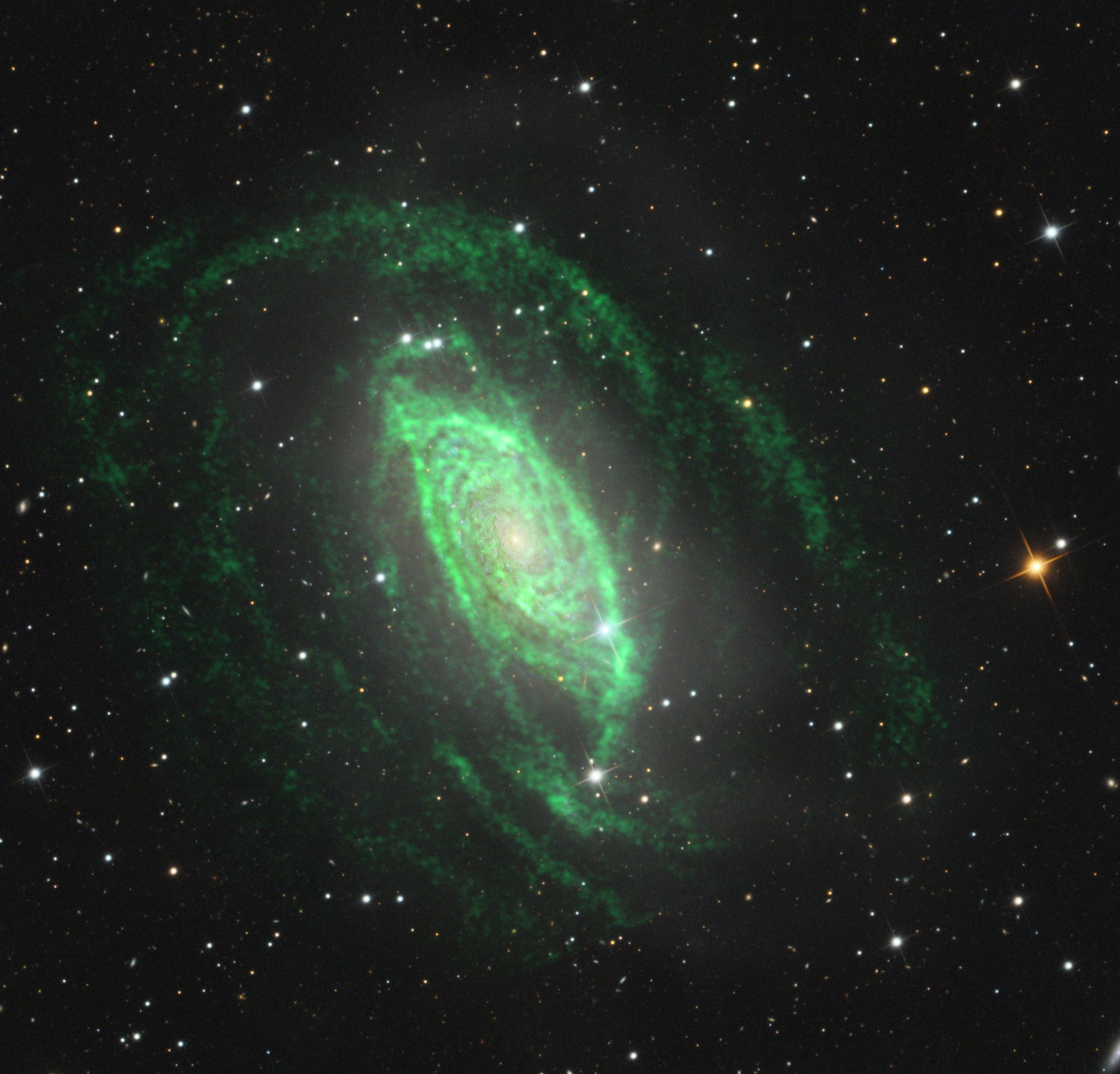
M63 HI Overlay
Other galaxies like NGC 891 or NGC 3351 have an even more enormous HI disk, stretching out to up to 4 times the visual radius of their host galaxy. This cold, atomic gas also takes part in the dynamics of a galaxy. In the image of M63 we can see, that it forms stellar streams (like observed in the optical) within the galaxy’s halo. These stellar streams are relics of ancient collisions of the galaxy with smaller satellites, that leave behind trails of ejected stars traveling along the former satellite galaxys trajectory. Furthermore, the HI disks follow the rotation of the optically visible stellar disk and can thus be used to measure the rotational velocity of the disk, helping in creating the popular rotation curves of spiral galaxies, which are one of the most important hints on the existence of dark matter.
In the form of high-velocity clouds, it hs been detected outside of the disk of many spiral galaxies, generally within ~ 10 kpc of the disk. It is estimated that about 25% of luminous spirals have more than 10^8 M_solar of atomic hydrogen gas outside the disk. In those cases where the HI mass is large it can be related to tidal features or streams indicating the accretion of smaller galaxies- like the Magellanic stream in our Milky Way, which contains most of the neutral hydrogen in our halo. [3]
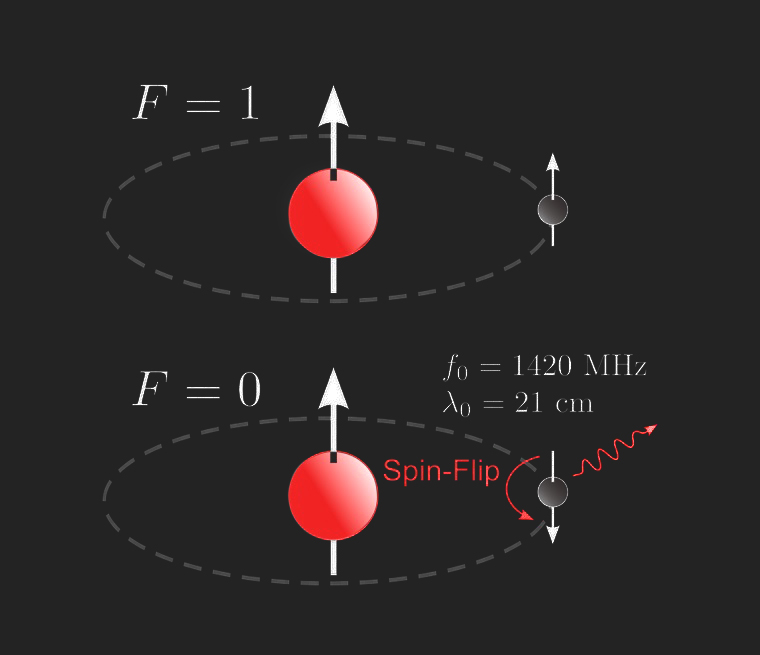
Atomic hydrogen and radio observations
Under athmospheric conditions on earth hydrogen exists as the diatomic molecular gas H2, in space however, conditions can be realized where the density is sufficiently low and cosmic radiation sufficiently high for hydrogen to occur as a single atom. This atomic hydrogen can be visualized by a single electron orbiting a proton. Both particles have an intrinsic quantum mechanical property called spin, which can either be oriented in one direction (“up”) or the other direction (“down”), but nowhere in between. When both spins are aligned in the same direction, the atom has slightly more energy than if they are aligned in opposite directions. When the atom changes its configuration from aligned spins to not-aligned spins, a small amount of energy is therefore released as radiation. This photon has a wavelength of about 21cm and can be observed via radio telescopes. Because of the longer wavelengths, radio telescopes have fuzzier vision than optical telescopes. To achieve the same resolution as an optical telescope, a telescope observing atomic hydrogen would need a dish about 40.000 times as large than the mirror of a conventional telescope. However, scientists found a way out of this problem by combining the signal of multiple dishes to achieve the resolution of a single large dish. This technique called interferometry, is nowadays commonly used to observe most sources in radio wavelengths. One of the observatories exploiting interferometry is the “VLA” (Very Large Array) in New Mexico, USA. It includes 27 radio dishes that can be moved on rails and be placed up to 21km apart, in order to simulate a larger dish. In 2007, this observatory was used to conduct the so called “THINGS” (The HI Nearby Galaxy Survey), which analyzed 34 galaxies in the milky way’s neighborhood and created images of the HI content of these galaxies for the exploration of the galaxies’ gaseous structure and dynamics closely related to the processes of star formation.
Overlay your astrophotos with HI survey data
Fortunately, this data is publicly available and can be easily accessed. So, if you want to see how the galaxy of your recent astrophoto looks in radio wavelengths or if you simply want to add another artistic layer to your photo - this is your chance! We compiled a list of steps you can follow to include this data in your images. It can be done using standard astronomy processing software and is here demonstrated using the common applications of Pixinsight and Photoshop CS6.
1. Access the data on the THINGS project webpage and check whether your galaxy is included in the THINGS survey using its NGC designation. Note that all Messier catalog galaxies are listed with their NGC number.
click here
2. Select and download the desired FITS file, clicking on either the “na” or “ro” labels in column “moment 0” of the table for your desired galaxy. Open the file in Pixinsight. Sometimes it can happen that it cannot be opened in Pixinsight due to a file error, this is solved by opening the file in a different software like “SAO DS9” or “AstroimageJ” and saving it again as a 16bit FITS file.
click here3. We need to align the HI image with your astrophoto. Since there are no stars in the HI image we can use to match the images, we need to help ourselves by a different approach of image alignment: “Alignment by Coordinates”. The Ra-Dec coordinates of the image, described by the so called “wcs” system can be used to align images without matching star positions. The HI image already has wcs coordinate information included, but the astrophoto might still need some. Therefore the Script “ImageSolver” (SCRIPT→ ImageAnalysis → ImageSolver) can be used. Entering the objects coordinates, the focal length and pixel size of your telescope, it should be able to plate solve your image and enter a wcs coordinate system in the file.
4. Once this is done, we can use the Script “AlignmentByCoordinates” (SCRIPT → Utilities → AlignmentByCoordinates). Once telling the script which images to align to each other it should work without further tweaking and we can save both images as 16bit TIFF files and continue to blend them in Adobe Photoshop.
5. The HI Image is placed as a layer above the astrophoto and blended in using the mode “screen”. Optionally one can, colorize the monochrome HI image in the color of choice to add a more artistic and dramatic effect to the blended image. Note that, if the alignment fails one can also quite easily align the images by hand since many dust structures are similar. If simply used for an artistic purpose the accuracy should be more than enough for a satisfying result.